Meet the divers trying to figure out how deep humans can go
Figuring out how the human body can withstand underwater pressure has been a problem for over a century, but a ragtag band of divers is experimenting with hydrogen to find out.
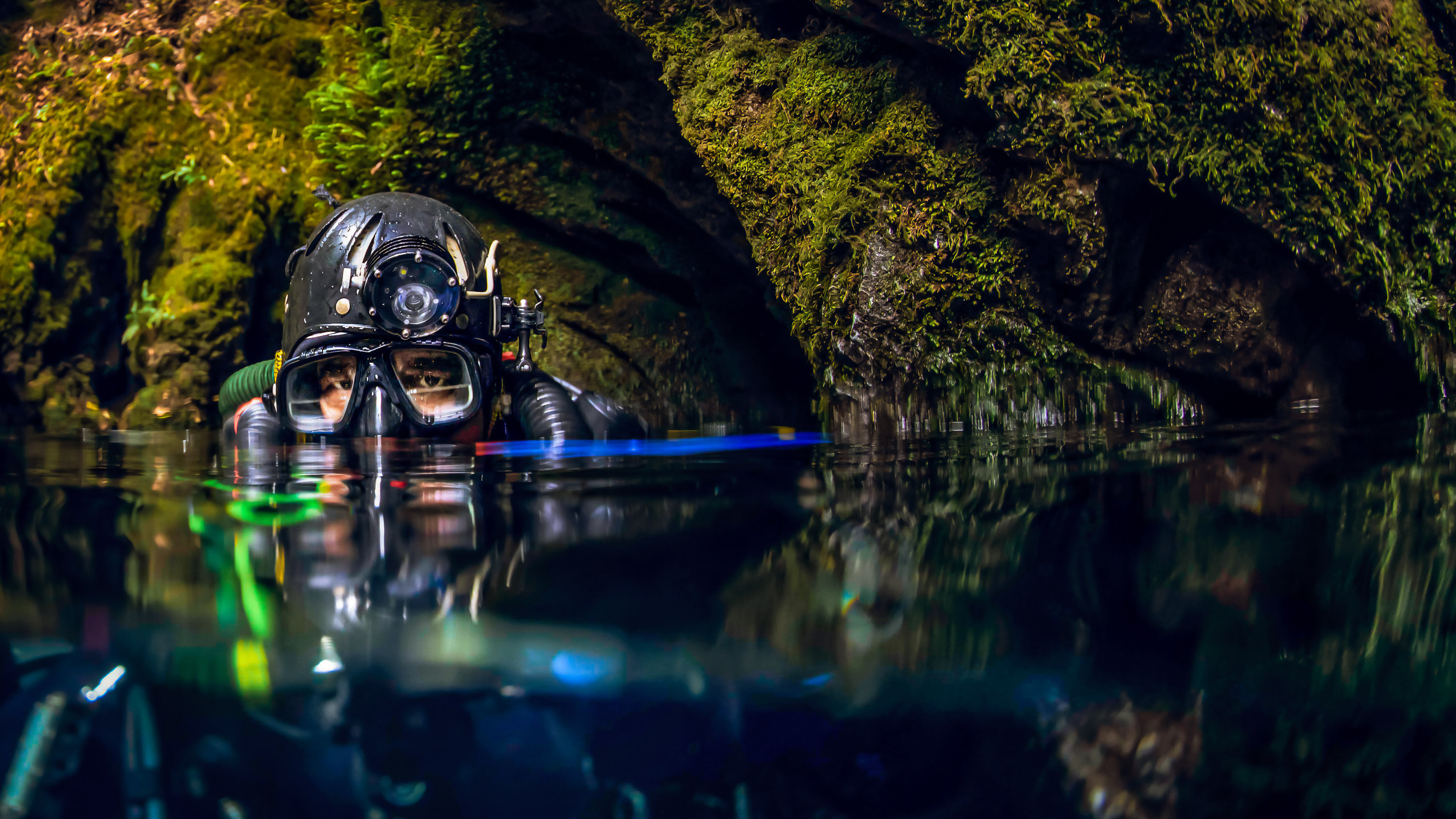
Two hundred thirty meters into one of the deepest underwater caves on Earth, Richard “Harry” Harris knew that not far ahead of him was a 15-meter drop leading to a place no human being had seen before.
Getting there had taken two helicopters, three weeks of test dives, two tons of equipment, and hard work to overcome an unexpected number of technical problems. But in the moment, Harris was hypnotized by what was before him: the vast, black, gaping unknown.
Staring into it, he felt the familiar pull—maybe he could go just a little farther. Instead, he looked to his diving partner, Craig Challen, floating a few feet to his right. They had both been diving increasingly dangerous and unplumbed caves for years, making them two of only a handful of people with the skills to assist in the rescue of the Thai soccer team that got trapped in one in 2018. They knew extreme risk, and each other, well. Even through the goggles and the mouthpiece of the breathing apparatus, its four thick hoses curling around his face like mammoth tusks, Harris could see that Challen felt the same way. They both wanted very badly to push forward into the dark expanse.
Instead, on Harris’s cue, they turned back. They weren’t there to exceed 245 meters—a depth they’d reached three years earlier. Nor were they there to set a depth record—that would mean going past 308 meters. They were there to test what they saw as a possible key to unlocking depths beyond even 310 meters: breathing hydrogen.
The problem has existed for more than a century: How can a human body withstand underwater pressure significantly past its natural threshold? Naval units and offshore oil companies around the world have long been invested in figuring it out for power and profit, and in the 1970s and ’80s their research began filtering into the civilian world, where people were testing the limits of their own curiosity.
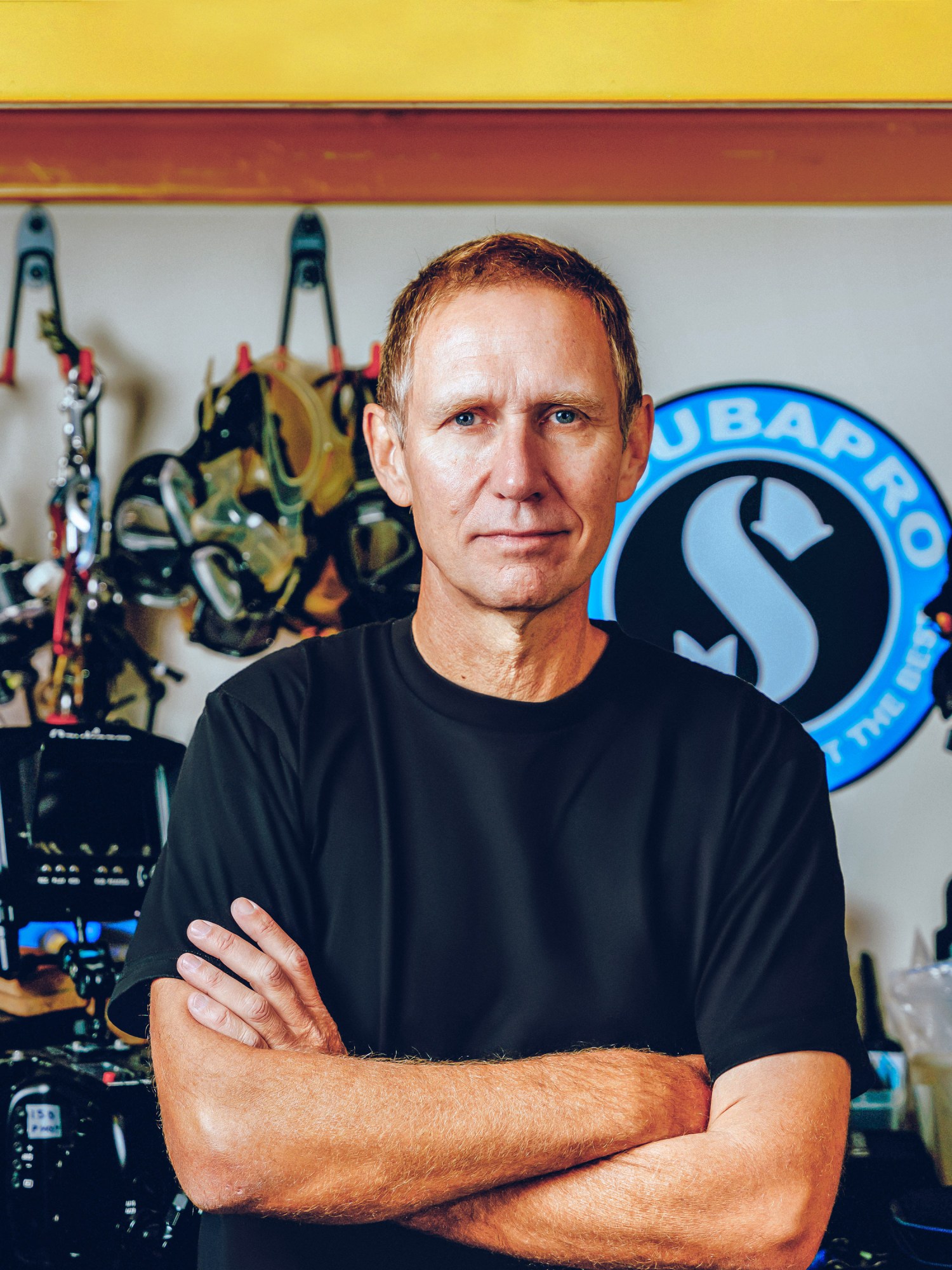
This included people like Sheck Exley, a high school math teacher in Live Oak, Florida. Exley became an international icon in the diving community for his record-breaking dives—exceeding, in some cases, the limits of the military and commercial professionals. He had been diving the underwater caves of North Florida since he was a teen—by 1972, at age 23, he was the first person in the world to log 1,000 cave dives—and exploring them is what pushed him to go deeper. He’d been hooked since his first cave dive in Crystal River, Florida, in 1966. “I kind of wandered off into the cavern there, my eyes adjusted, and I swam a little bit further, peering off into the darkness,” he told AquaCorps, a magazine for divers, in 1992. “I guess I’ve been peering off into that darkness ever since.”
At around 40 meters, breathing the gas mixture we call air—78% nitrogen, 21% oxygen, and 1% trace gases—causes inert gas narcosis, or the “martini effect,” named for the incapacitating state it induces. A little deeper and oxygen becomes toxic. For years, the US and British navies had been using helium to dilute the oxygen and nitrogen in a diver’s tank as a means of counteracting both these problems, but few outsiders knew about it. In 1981, after the German cave diver Jochen Hasenmayer reached 143 meters using a helium mixture, Exley started using it as well—despite the knowledge that only a few years earlier, two divers in Florida had experimented with the mixture and died.
Divers who go past 40 meters typically don’t use a constant gas ratio—they cycle through mixes of nitrogen, oxygen, and helium as they descend and ascend, modifying them according to location, water temperature, neurological tolerance to narcosis, and many other variables. Decompression tables, which lay out different gas mixtures and the amount of time to be spent breathing them, provide a precise road map for this process—very necessary, as ascending too quickly releases the accumulated gases in a diver’s blood and tissue the way unscrewing the cap on a bottle of soda releases bubbles, resulting in the painful, often debilitating condition known as the bends. Without the tables, ascending from great depths was too risky. But generating them required high-level technology and archives of data unavailable to most people.
Exley was able to persuade a friend who worked in commercial diving to give him a sample table, which he used to extrapolate past 121 meters, working everything out on his computer. In 1987, guided by this information, he used helium to successfully break the 200-meter diving barrier at Nacimiento Mante in Mexico—a 24-minute descent that forced him to stay underwater for 11.5 hours to decompress. Over that period, he felt himself becoming dangerously weak. His blood sugar dropped, the cold seeped into his limbs, and his exposed hands and face began to grow wrinkly, become raw, and then flake. “At the time I felt I could have dived deeper,” he told InDepth magazine that year. “But I knew that I had reached my decompression limits.”
In 1988, Exley beat his own record, hitting 237 meters using a more precise table generated by the physiologist Bill Hamilton. Known among divers as “The Prince of Gases,” Hamilton had been involved with some of the earliest work on decompression past 200 meters.
He had helped develop a computer program that generated extremely precise tables calibrated to any number of parameters, which he created for commercial diving companies and navies around the world. “Through them, he had staggering amounts of data,” says Bill Stone, an aerospace engineer and cave diver who built and sold an early rebreather—a type of breathing apparatus that recycles exhaled air by scrubbing it of carbon dioxide. “He processed all that and developed stochastic models that figured out where you could gain speed on decompression and not hurt yourself.” In the ’80s, Hamilton made the unprecedented move of creating custom tables for people who were not commercial or military divers, forever endearing him to the diving community.
But in 1994, Exley died attempting to reach the bottom of the Zacatón sinkhole in Mexico, 332 meters down—he got to 270 meters. A likely factor was high-pressure nervous syndrome (HPNS), a neurological condition involving uncontrollable tremors brought about when a diver descends too rapidly at high pressure past 150 meters. Adding nitrogen to the mix of helium and oxygen could help—nitrogen’s narcotic effects could dampen the symptoms. But at that depth, adding more nitrogen would make it more difficult to breathe because of the gas’s density, and the narcosis would be debilitating.
Divers still pushed past Exley’s record into 250 and then 300 meters, white-knuckling through the HPNS symptoms. But at such depths, helium becomes too heavy for the human body to process. “As you go deeper, for every 10 meters you get an extra atmosphere of pressure—so once we’re down at those depths of 250 meters, that’s 26 atmospheres,” Challen, Harris’s dive partner, told me. “It becomes a physical problem of moving the gas in and out of your lungs.” In order to alleviate this problem, divers would need to breathe something lighter than helium.
“And we’ve only got one lighter gas to use,” Challen says. “After hydrogen, there’s nothing.”
What became known as the H2 Working Group began as a “thought experiment,” according to Michael Menduno, a veteran diver and editor in chief of InDepth magazine, who brought everyone together in collaboration with John Clarke, the former scientific director of the US Navy Experimental Dive Unit. It was also a way to socialize the way scientists, engineers, and tinkerers know how: by arguing over how to make something work.
The idea for the working group emerged in 2020, when Menduno, while researching an article on deep dives past 250 meters, concluded that the diving world had reached its limit with helium. Exley himself had pointed to what he saw as the next step in reaching unprecedented depths: “From what I’ve been learning, there seems to be some real potential for hydreliox”—that is, an oxygen-helium-hydrogen mix. Still, “no one has looked at its use for deep bounce dives,” he told Menduno in a 1992 interview, referring to dives without the use of a chamber to help the diver reach a safe gas saturation point before venturing into the water.
When the group formed in that year, at the beginning of the pandemic, Menduno was familiar with previous attempts to use hydrogen. In the ’90s he had traveled to France to watch a team of medical doctors, engineers, scientists, and trial divers from Compagnie Maritime d’Expertises (Comex), a Marseilles-based company focusing on deep diving systems and equipment, attempt to pass 701 meters in a hyperbaric chamber pumped with a hydrogen gas mixture. (It required 15 days of compression and 23 days of decompression.) This was Hydra 10, part of a series of experiments using hydrogen. After Hydra 12, in 1996, the project had scrapped pursuing hydrogen altogether. Companies were turning to manned underwater vehicles and saturation diving—that is, staying at extreme depths long enough to allow the inert gas to fully dissolve in a diver’s blood, which is only possible with a chamber. “[In commercial diving] there’s no poetry, no sense of humor—you just want to be efficient,” says Jean-Pierre Imbert, who worked on the Hydra project and had invited Menduno to observe the experiments in the ’90s.
At 40 meters, the gas mixture we call air becomes incapacitating. A little deeper and oxygen becomes toxic.
In 2012, building on Comex’s work, a team of Swedish divers, called the Hydrox Project, had successfully breathed a mixture of hydrogen and oxygen for five minutes at 40 meters. They were hesitant to share the information, concerned that people without the expertise might make the attempt at their peril. “We didn’t publish or tell too much about how we did it, because we believe if you do this wrong—you have to understand it, or you will fail miserably,” diving engineer Åke Larsson, one of the members of the Hydrox Project, later said. “But it’s not rocket science. If you do your homework, it’s not complicated. But you have to do it right.”
That was the history on Menduno and Clarke’s minds when they began calling up experts in the field to discuss whether it would be possible for a diver outside a chamber to descend beyond 200 meters breathing hydrogen. Each member was an expert in a different element of what would be involved in such a dive—the mechanics of the breathing apparatus, the calculation of gas mixtures, the physiological issues to be overcome as divers coped with cold and pressure. By the end of the first meeting, they had come up with so many potential problems they created what they called a “challenge tree” to organize them; members gravitated toward their areas of expertise and came back to present possible solutions to the group. The tree kept branching; solutions begat new problems.
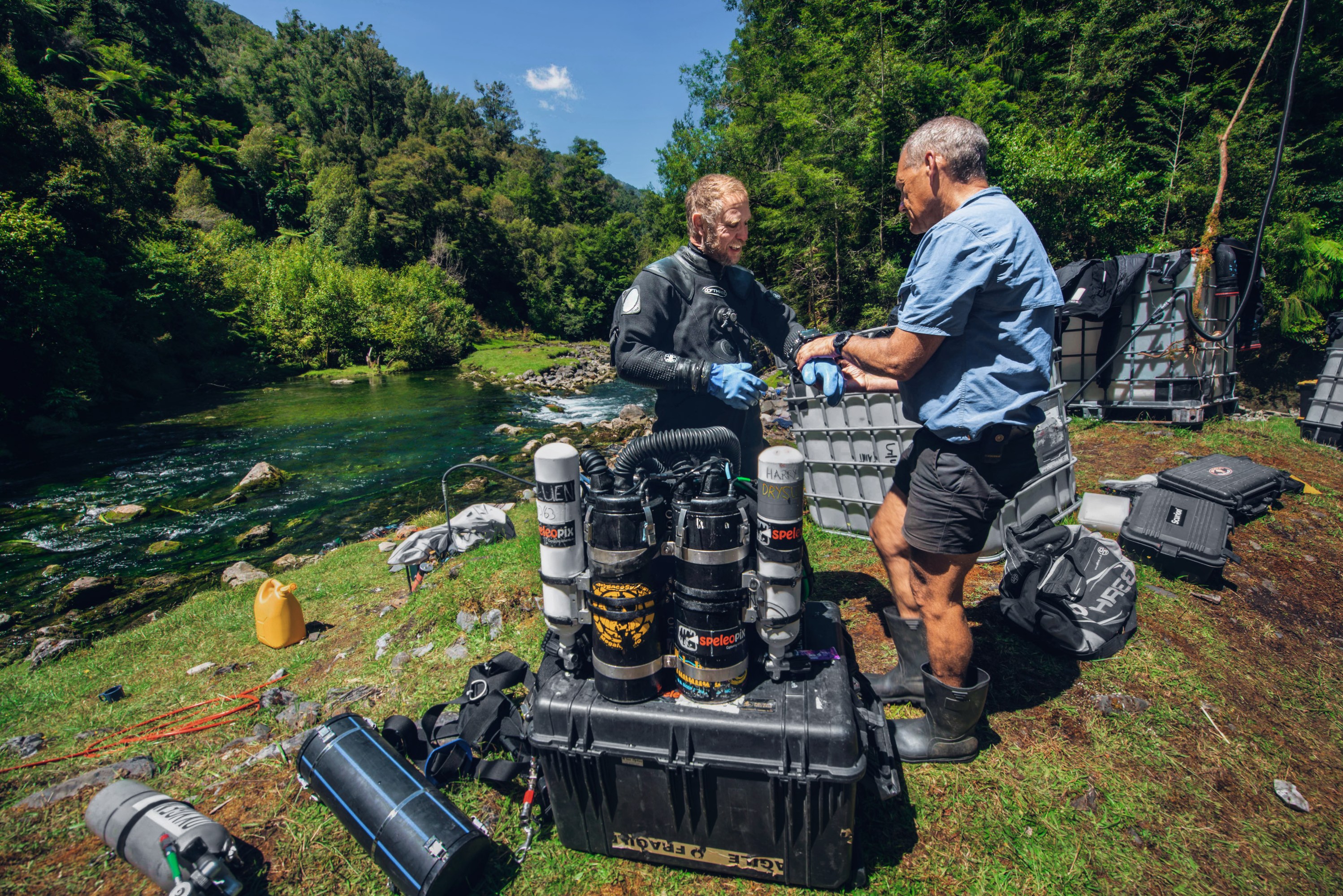
Harris joined the working group at Menduno’s invitation. The team of divers he was a part of, the Wetmules, had reached 245 meters at the Pearse Resurgence, the source of the Pearse River in New Zealand, but the cave stretched on, and they wanted to map it. They knew that helium was not going to get them past 300. Hydrogen, however, was nerve-racking. “I don’t really want to be the guinea pig who famously popped under water after switching to hydrogen,” he told the group at the first meeting. “So it’d be nice if we can solve that.”
Hydrogen—as the Hindenburg disaster showed the world—is highly flammable. A spark hundreds of times smaller than the slightest amount of static you can feel on your fingertip would be enough to ignite it. And even if such a spark didn’t cause an explosion, it could still set the gas on fire. “If you’re breathing that mix when it’s burning,” Clarke told the group, “it’s going to be a very unpleasant dive.”
David Doolette, a research physiologist at the US Navy Experimental Diving Unit who had himself dived the Pearse Resurgence in the ’90s, was skeptical but considered himself “dihydrogen-curious,” referring to the molecular structure of hydrogen gas. For one thing, its thermal properties mean divers risk hypothermia. “It’s gonna suck the heat out of your body to a dangerous level,” he told the group. And there was no data available to calculate the appropriate decompression times. “It’s going to be a challenge to do the decompression calculations,” he told the group. “Well, not a challenge doing that—that’d be easy. It’d be a challenge to get them right so that they were safe to dive.”
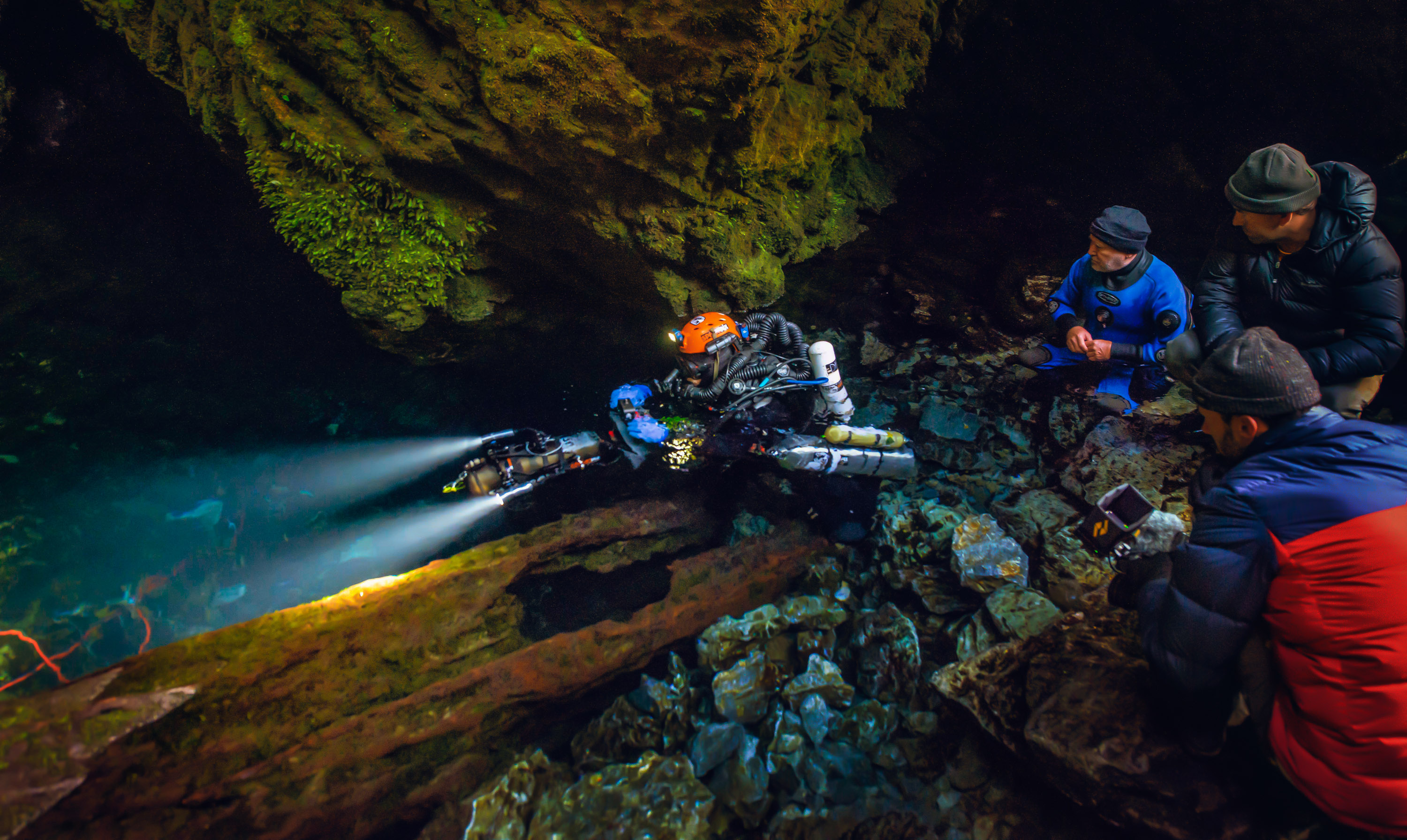
Others shared his skepticism. Nuno Gomes, a former world-record-holding cave diver, was particularly concerned about decompression. “I think if we’re going to go with this, we’re going to have to progress slowly,” he told the group. “Start with shallow dives and progress to deeper and deeper dives.” But according to Doolette and Stone, the aerospace engineer, it soon became clear that Harris had already decided to try hydrogen in the Pearse Resurgence. At a conference in Australia in 2022, as members of the H2 Working Group met up to talk in person, Imbert said that going beyond 3.5% hydrogen would likely trigger a detonation.
“Harry nods and goes, ‘Well, I don’t think that’s true,’” Stone told me. Imbert asked how he could prove that. “Harry says, ‘Well, last week I did 7% in my pool.’ Everybody perked up.”
Harris had ordered a canister of hydrogen delivered to his suburban home in Adelaide and, as he later explained, “decided to have a bit of a play with it.” He rigged his rebreather for hydrogen and put it in his backyard pool, hoping to contain any potential blast. He filled the rebreather with hydrogen and then, backing way from the pool, began to introduce oxygen. (His dog observed from outside the pool fence; his wife was out.)
When nothing exploded, he started adjusting the ratio of oxygen and hydrogen, becoming confident enough to try using the rebreather himself. His first sip, he later told me, felt light, slippery, and cold. It was almost delightfully easy to breathe. “Hydrogen voice is much sillier than helium voice,” he told me. “And I was pleased the house and the dog were intact.”
The others were amazed. Some were perturbed. “Everybody has to make this decision for themself,” Stone told me. “The Pearse Resurgence is not a place to experiment. When you go in there, you should be using gear and techniques that you know are going to work at that depth. You don’t want to be doing physiological experiments at 300 meters’ depth. That’s what killed all of the other divers who went beyond 200 meters’ depth. So my advice to Harry and anybody else who wants to play this game is the same as what I gave Exley: Go. To. A chamber. Simulate this first.”
“The group was sort of split,” Menduno told me. “I mean, everybody was supportive of Harry, but there were some people in the group that thought: You’re gonna die. Some of the people in the group were upset and worried that their friend was going to go off and do this thing and potentially die.”
Around the first corner of the Pearse Resurgence, the light disappears, as though the dark walls, black marble striated with veins of gray quartz, have absorbed it. The cave sometimes narrows so much that if you stood, you could touch the ceiling. Other parts billow out into enormous chambers. At one point, jagged fingers of rock bristle from the walls. Other, deeper parts of the cave are smooth and almost perfectly round, broken only by dark fissures that lead to unexplored tunnels.
As each section of the cave gets discovered, it receives a name. Going down in February 2023, Harris and Challen passed through the Nightmare Crescent, the Needlebender, the Gargleblaster, Weaver’s Ledge, the Big Room, and finally the Brooklyn Exit. The water was 6 °C and perfectly clear. Aside from the brief hisses and clicks of the rebreathers—the crackle of the solenoid triggering, the sigh of gases being pumped through the loop—there was an otherworldly silence.
At 120 meters, the cave opens up onto a plateau that drops off into an abyss. “At that point it’s like standing on the precipice,” Harris told me. “And it feels like you are really beginning the journey.”
The abyss takes you down 50 meters through a vertical tunnel. By 170 meters, Harris could track where he was on the map in his head, following familiar rock formations. They wanted to preserve their energy and prevent carbon dioxide buildup in their joints, so they limited their movement, relying on underwater scooters to move. They slowly tied off at different points in the descent, working around ropes left behind from dives past, some of which had been installed by Doolette 20 years before.
At 230 meters, Harris had done something nobody had done before—swimming freely to unimaginable depths and breathing in hydrogen.
Harris remembers that even though his mind was absorbed with their strict plan, hypervigilant to any strange noises from his rebreather that could mean failure, he took a moment to pause, thinking: “What if I never got to see this again?”
At 200 meters, Harris introduced the hydrogen. For the next 30 meters he gauged his body’s reaction. He was calm, clearheaded, but even more, he noticed that the light tremors in his hands he usually got at this depth, an early sign of high-pressure nervous syndrome, had disappeared. He looked to Challen, who was using helium, as he tied off the rope: his dive partner’s hands had a visible tremor.
At 230 meters, Harris had done something nobody had done before—swimming freely down to these unimaginable depths and breathing in hydrogen—but his eyes were trained just a little farther, to the unexplored drop only 15 meters away. “I would be lying if I didn’t say I dream about going down there,” he later told an audience in Malta.
Those obsessed with learning how to put the human body under incredible amounts of pressure make up a relatively small community; for the most part, everybody is one degree of separation from everyone else. Clarke, the Navy scientist, and Susan Kayar, a physiologist and expert in decompression who was also part of the H2 Working Group, had both worked at the Navy Medical Research Institute in the ’90s. They had lost touch, but they reconnected after both published novels that included a scene with a hydrogen dive—hers for a rescue mission, his to recover a UFO that had crashed into the sea. “Hers is more realistic in that regard,” Clarke says. “I hadn’t spoken to Susan in 30 years, and then we finally found out: Gee, we’re thinking very much alike!”
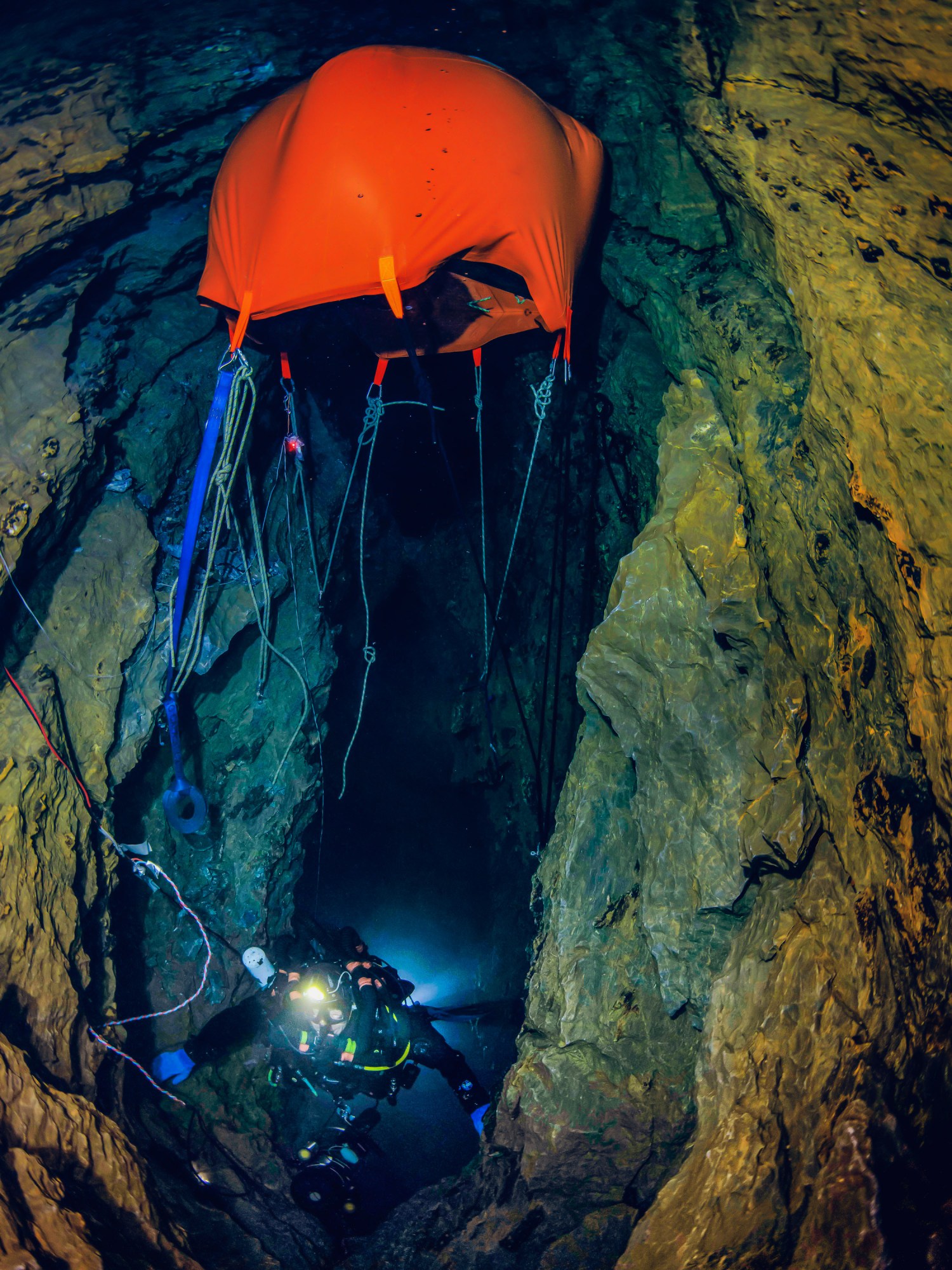
Doolette, the research physiologist skeptical about using hydrogen, has known Harris for more than 20 years, ever since Harris took Doolette’s diving medicine qualification course in Australia. At the time, they were also both working in the hyperbaric medical unit at the Royal Adelaide Hospital. Harris wanted to get more advanced at cave diving and Doolette was a longtime technical cave diver.
Even before the H2 Working Group began meeting, Doolette knew that Harris was thinking about diving with hydrogen. “I knew things were being planned,” Doolette told me. “It probably came as a surprise to some of the workshop, but not to me … I knew it wasn’t theoretical.”
But Doolette felt that testing hydrogen on a deep cave dive was too risky. “There’s a whole hydrogen industry—and their approach is to exclude hydrogen and oxygen from getting near each other. So when you’ve got to mix them together—well, there aren’t even procedures, really,” he says. “I certainly thought it was foolhardy.”
Stone, too, was skeptical of the expedition—for any dive beyond 200 meters, he said, the concept should be tested first. “I’ve been doing this for a long time, and I’ve got a lot of dead friends,” he says.
“We’re moving into the zone where robotics isn’t science fiction,” he continues. “It’s hardware, it’s software—we’re doing it. Is it perfect? No. But it’s a couple years away. We’re close to doing dives longer than the longest human dives, and with a kilometer-depth rating it’ll be far, far beyond even hydrogen diving.”
As a diver, Doolette says, he understands wanting to go where nobody else had been before: “Cave divers and other people explore for the joy of it. A robot’s not gonna get that.” The other draw, he says, is the puzzle of figuring out where the cave is going and how to follow it. And when you finally get into the water, the rest of the world falls away.
A few weeks after the hydrogen dive, Harris gave a presentation to the H2 Working Group, apologizing for “sneaking around a little bit.” He concluded with a caveat: n = 1. “Meaning it has been successful one time,” he said. When the PowerPoint ended, the group applauded. “Just something you can add to your final slide, Harry,” Doolette said. “The probability of survival is greater than zero.”
Doolette was relieved when he learned that Harris had made it back to the surface. “I recognized that it was a pretty groundbreaking, momentous dive,” he told me. “I don’t see the purpose of going deep for the sake of going deep, but if you’re committed to an exploration project and it involves cave diving in pursuit of exploration and discovery, then that’s what you do.”
“There’s always a little temptation to go that little bit further. That’s why we’re doing this stuff,” Challen told me. “We have this defective aspect to our characters that urges us to push on just a little bit more.”
Samantha Schuyler is a writer, editor, and fact-checker living in New York.
Keep Reading
Most Popular
Large language models can do jaw-dropping things. But nobody knows exactly why.
And that's a problem. Figuring it out is one of the biggest scientific puzzles of our time and a crucial step towards controlling more powerful future models.
OpenAI teases an amazing new generative video model called Sora
The firm is sharing Sora with a small group of safety testers but the rest of us will have to wait to learn more.
Google’s Gemini is now in everything. Here’s how you can try it out.
Gmail, Docs, and more will now come with Gemini baked in. But Europeans will have to wait before they can download the app.
How one mine could unlock billions in EV subsidies
The Inflation Reduction Act is starting to transform the US economy. To understand how, we tallied up the potential tax credits available as the nickel from a single mine flows through the supply chain.
Stay connected
Get the latest updates from
MIT Technology Review
Discover special offers, top stories, upcoming events, and more.